Future planned missions to the Moon and Mars rely on in-space assembly and other methods to make ambitious habitats possible. Constructing large structures in the microgravity environment of space costs a fraction of the cost of building on Earth and launching them. Ongoing maintenance will likewise require innovative methods, such as additive 3D printing in orbit.
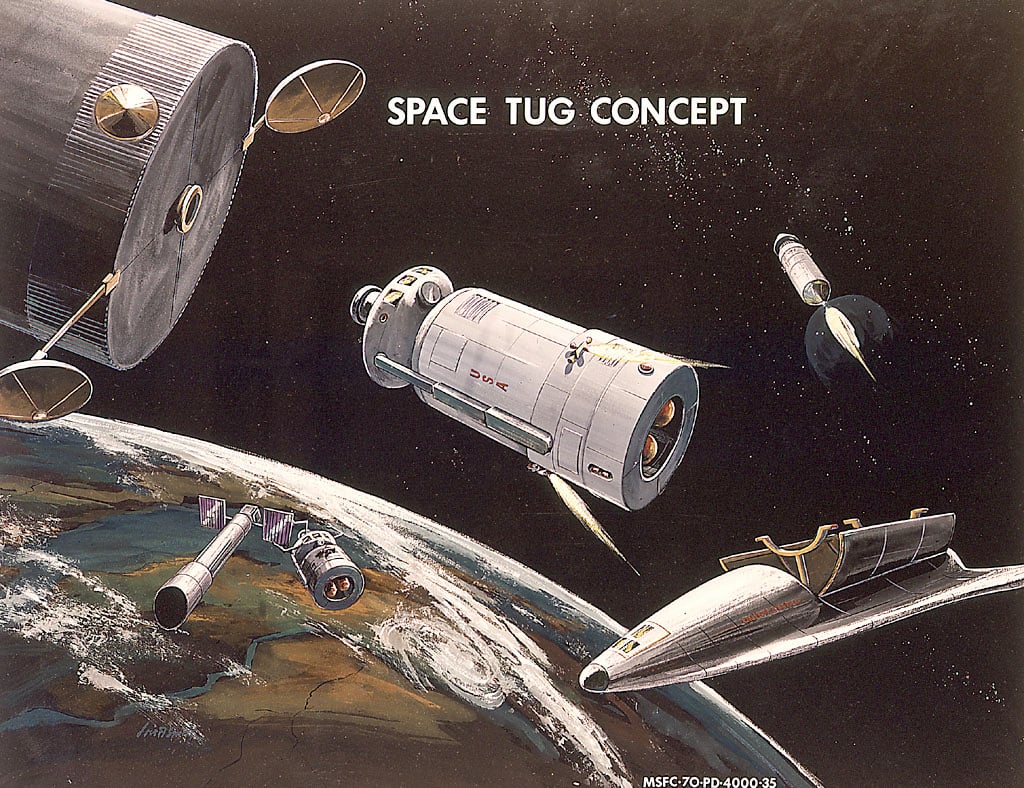
NASA ID: 9902041
Managed by Marshall Space Flight Center, the Space Tug was intended to be a reusable multipurpose space vehicle designed to transport payloads to different orbital inclinations. Utilizing mission-specific combinations of its three primary modules (crew, propulsion, and cargo) and a variety of supplementary kits, the Space Tug would have been capable of numerous space applications. This 1970 artist’s concept illustrates a Space Tug Concept, crew module attached, in conjunction with other space vehicles. The Space Tug program was cancelled and did not become a reality.
Quick Facts
- Robotic arms and autonomous robots are key technologies for in-space assembly.
- The International Space Station (ISS) is an example of a structure assembled in space.
- NASA’s On-orbit Servicing, Assembly, and Manufacturing (OSAM) initiative aims to develop technologies for in-space construction.
- 3D printing in space can produce components on-demand, reducing the need for spare parts to be launched from Earth.
- The Lunar Gateway, part of NASA’s Artemis program, will be assembled in lunar orbit.
- In-space assembly can reduce launch costs by allowing smaller, more efficient rockets to be used.
- Space tugs are being developed to assist with the positioning and assembly of space structures.
- Solar panels and other large structures can be more effectively deployed and maintained through in-space assembly.
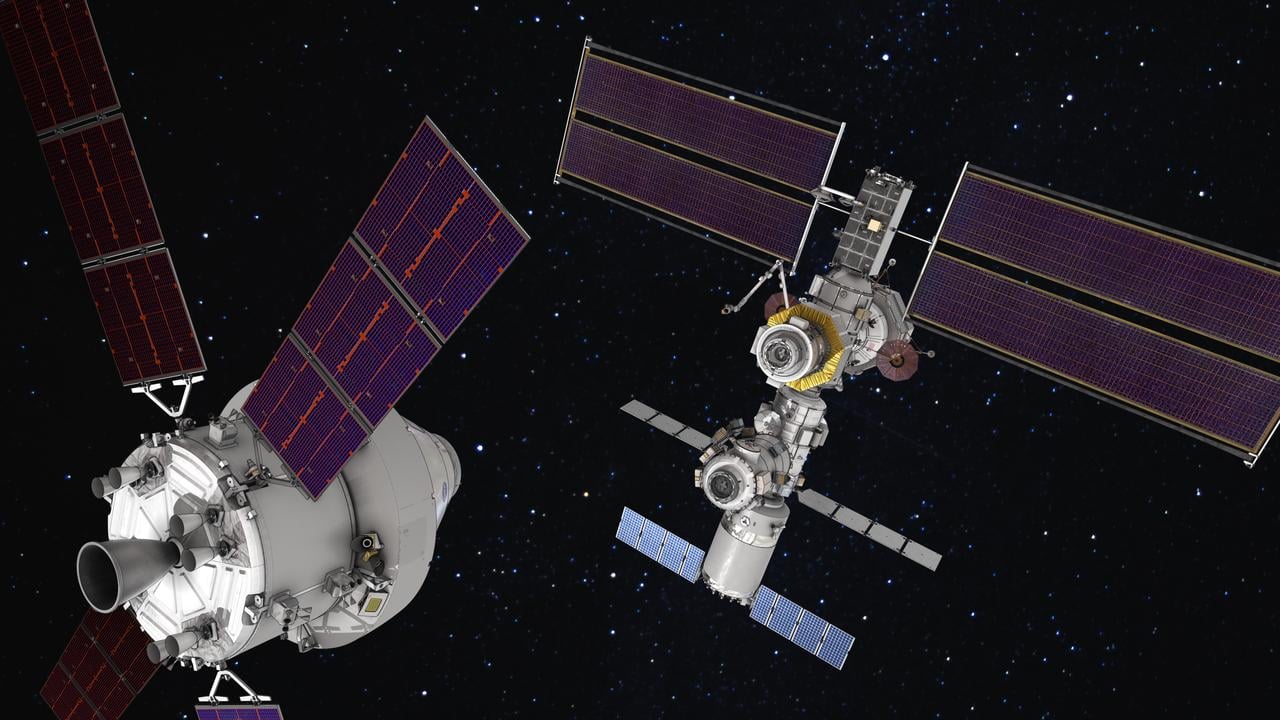
NASA ID: Gateway-Telephoto-IAC7-Set-4k-BG_4
The Gateway space station will be humanity’s first space station in lunar orbit as a vital component of the Artemis missions to return humans to the Moon for scientific discovery and chart a path for humans to Mars. A modular approach with components delivered and assembled in space will make this project efficient and feasible.
Autonomous and Semi-Autonomous Robotics
Several key technologies are driving this innovation forward. One of the foundational elements is robotics. With the ability to operate autonomously or semi-autonomously, robotic systems are essential in the harsh and unpredictable environment of space. From assembling satellite modules to constructing entire space habitats, robots are proving indispensable.
The advantages don’t stop there. Imagine the flexibility and cost savings when materials can be delivered in smaller, more manageable shipments and assembled in orbit. This approach reduces the need for massive rockets and mitigates the risks associated with heavy launches.
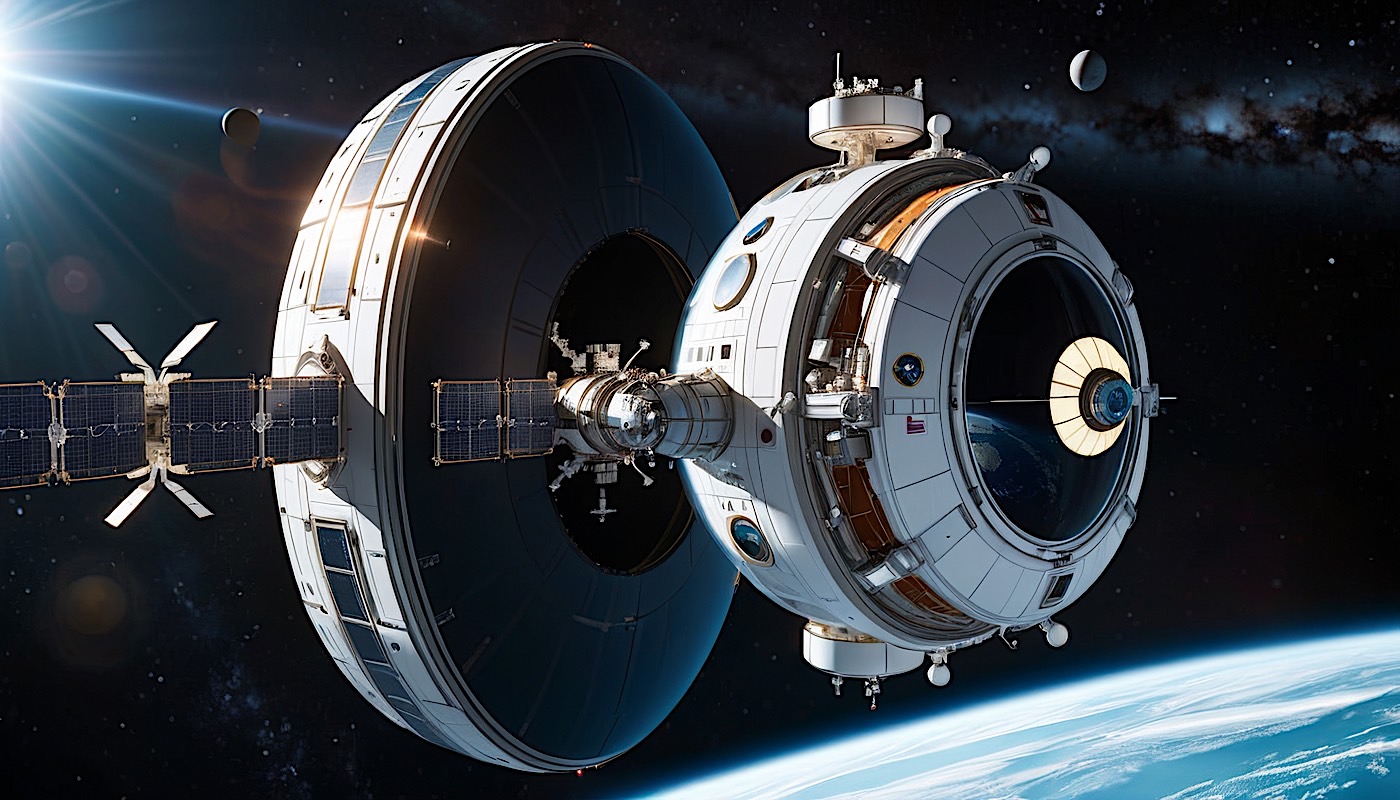
Key Technologies
Key technologies empowering in-space assembly and construction include:
- Robotic Arms and Manipulators: These are critical for precise movements and assembly tasks. Robotic arms like the Canadarm2, used on the International Space Station (ISS), demonstrate how effectively they can handle docking and maintenance operations.
- Autonomous Drones: These can inspect and repair structures, providing real-time feedback to mission control centers on Earth.
- 3D Printing: Additive manufacturing technologies allow for the creation of components directly in space, reducing the need to transport fully-finished parts from Earth.
- Modular Design: This involves creating spacecraft and habitats out of pre-fabricated modules that can be easily assembled in orbit, enabling rapid construction with fewer resources.
These technologies, working in concert, make the dream of permanent lunar bases, Martian colonies, and massive space telescopes a tangible reality. As you explore these innovations, you’ll see how each contributes to a more efficient and sustainable way of venturing into the cosmos.
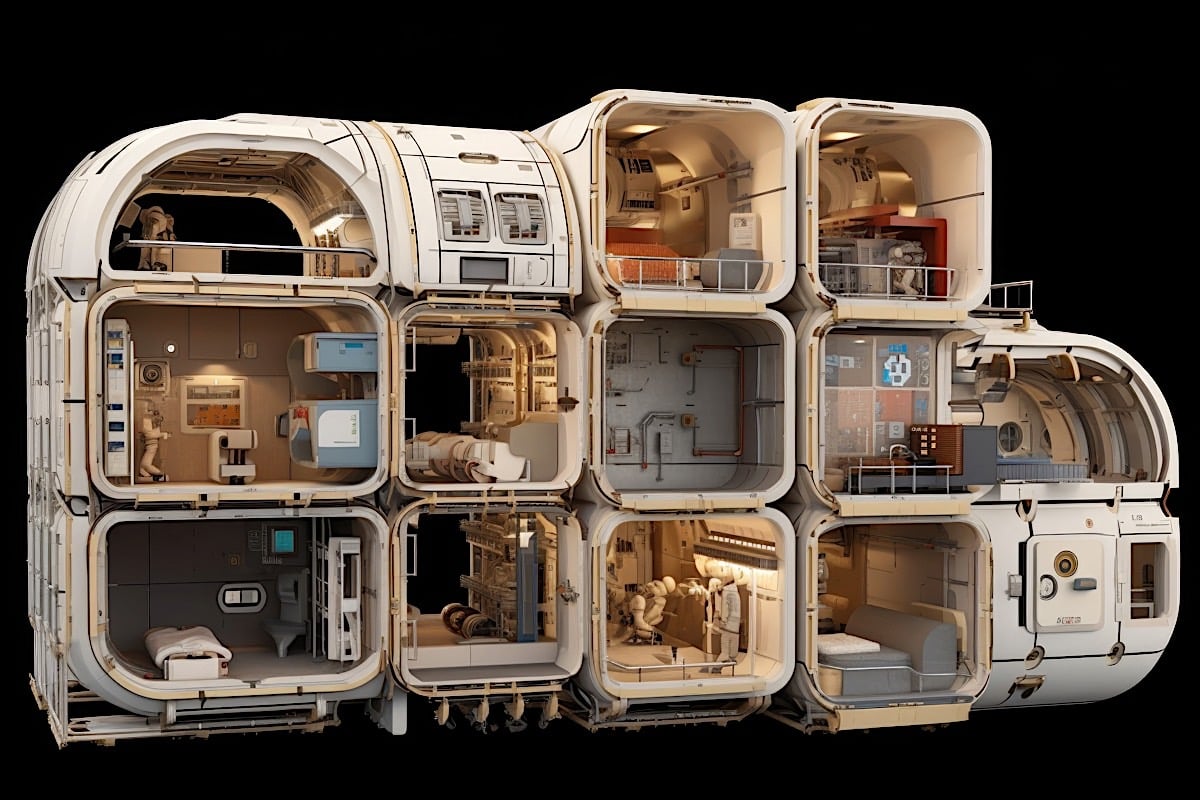
Quick Stats
- The International Space Station (ISS) was assembled in orbit over 13 years with contributions from 15 nations
- The cost of launching materials into space is approximately $10,000 per pound
- Robotic arms like Canadarm2 have been used for over 20 years for in-space assembly tasks
- SpaceX’s Starship aims to carry over 100 tons of cargo to Mars
- The Lunar Gateway is planned to be a modular space station in lunar orbit, with initial modules launching in the 2020s
- NASA’s OSAM-1 mission will demonstrate satellite servicing and assembly in orbit
- The European Space Agency’s (ESA) Bartolomeo platform allows for external payload hosting on the ISS
Modular Design: Building Blocks for the Final Frontier
Modular design isn’t just a buzzword; it’s a transformative approach that underpins many contemporary and future space missions. The essence of this concept lies in creating structures composed of interchangeable modules or components. This allows for greater flexibility, easier repairs, and significant enhancements over time. Imagine space habitats with segments that can be swapped or upgraded, adapting to the evolving needs of astronauts and mission requirements.
The International Space Station (ISS) is a stellar example. Each module serves a distinct purpose, and the ability to connect new modules extends its functionality. These modular structures make construction less daunting, cutting down the complexity of launching fully integrated systems. In essence, supplies and new technology can be incrementally included, reducing risks and costs while improving adaptability.
Beyond simple adaptability, modular design encourages collaborative international missions. Various countries can contribute different modules, fostering international cooperation. Moreover, this method paves the way for scalability. Need more living quarters as the crew size expands? Just add another segment. Planning a research mission that requires specialized equipment? Attach a new lab module.
Modular design also plays a vital role in the prospective construction of lunar bases or Martian habitats. By sending smaller, manageable parts ahead of human arrival, we can automate initial setup. Robots or rovers could assemble these modules, creating a sustainable and habitable environment even before astronauts set foot.
Clearly, the building blocks for the final frontier are not monolithic structures but versatile, adaptable modules. This ensures not only the success and sustainability of future missions but also opens the doors to endless possibilities in how our off-world habitats will evolve.
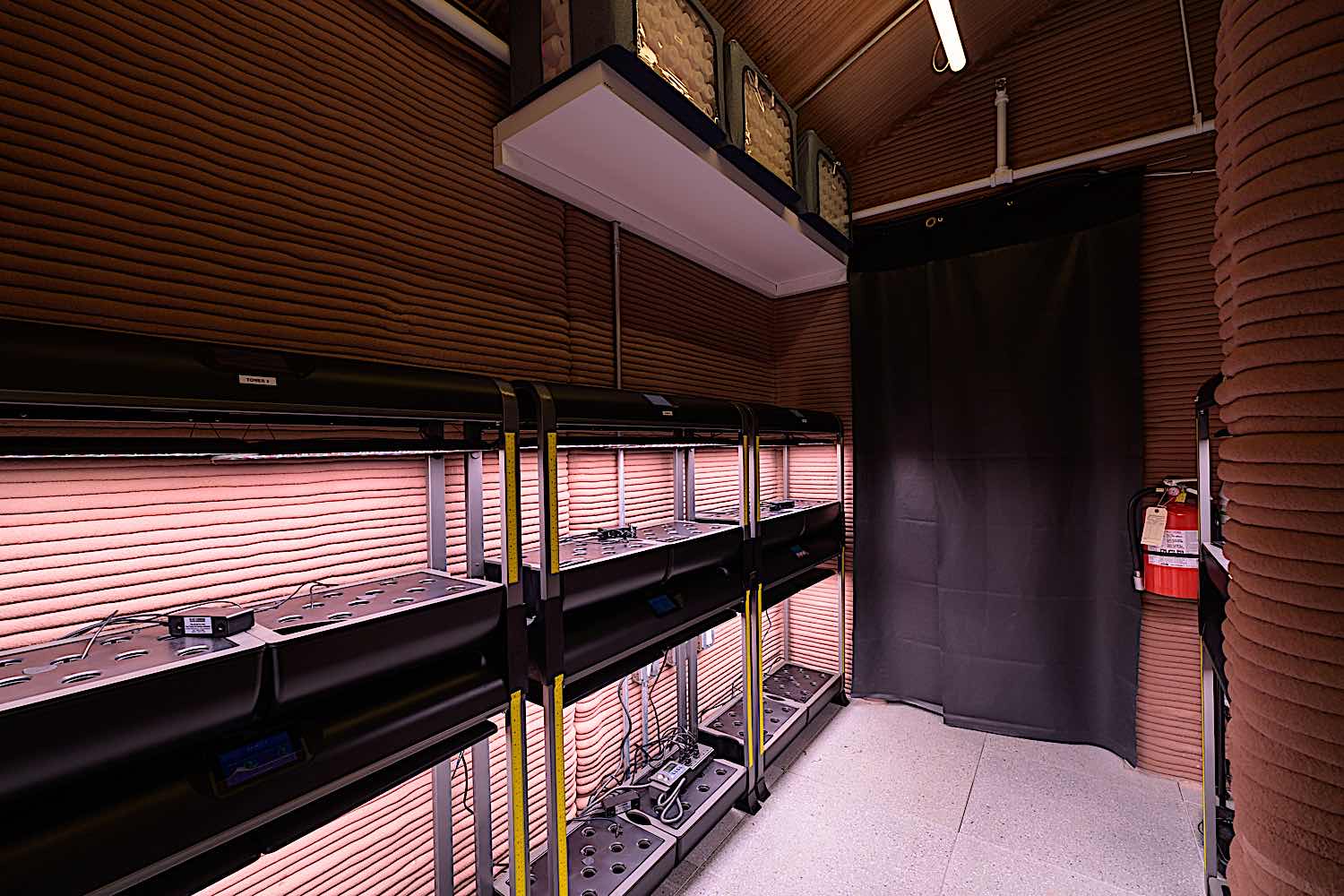
3D Printing in Space: Crafting the Future Off-Earth
- NASA’s 3D-printed habitat challenge aims to develop sustainable housing solutions for space exploration.
- 3D printing can utilize in-situ resources like lunar or Martian regolith, reducing payload weight from Earth.
- SpaceX’s Dragon spacecraft has deployed 3D-printed components, exemplifying current real-world applications.
- Advanced 3D printing techniques are capable of producing complex structures that are lightweight yet incredibly strong.
- Astronauts aboard the International Space Station (ISS) already use 3D printers to create tools and spare parts on-demand.
- Future missions plan to use a mix of robotic assembly and 3D printing to construct bases on other planets.
Cost-Effective Exploration: The Economic Advantages of In-Space Assembly
Aspect | Traditional Methods | In-Space Assembly |
---|---|---|
Launch Mass | High | Reduced |
Cost | $10,000-$20,000 per kg | $1,000-$10,000 per kg |
Flexibility | Low | High |
Risk of Failure | High | Reduced |
Assembly Time | Months to Years | Days to Weeks |
From the table above, it’s evident that in-space assembly can dynamically transform the landscape of space exploration. You see significant reductions in launch mass and cost, both of which are pivotal in making space missions more sustainable and efficient. Traditional methods demand heavy payloads, leading to skyrocketing expenses—ranging between $10,000 and $20,000 per kilogram launched. Contrast that with $1,000 to $10,000 per kilogram for in-space assembly, and you get a clearer picture of the economic advantage.
Flexibility is another game-changer. Traditional approaches are rigid and unforgiving, plagued by the significant risk of failure that comes with the territory. In contrast, the modular and adaptable nature of in-space assembly vastly reduces this risk, allowing for more robust mission plans and quicker adjustments. Imagine constructing a space habitat swiftly and efficiently, within days to weeks, as opposed to the protracted months or even years required through conventional approaches.
The implications extend beyond cost and efficiency. As the space environment is an inherently harsh and unpredictable frontier, the ability to deploy and reconfigure components with high flexibility and minimal risk is a monumental step forward. This adaptability opens the door to more complex, ambitious missions—possibly multi-phase explorations that evolve over time.
The enhanced efficiency in assembly time cannot be overstated. Rapid construction timelines empower new missions to pivot and respond to unforeseen challenges. You’re essentially looking at a revolution in how we plan, build, and sustain our off-world endeavors, setting the stage for more daring, data-rich, and ultimately successful missions.
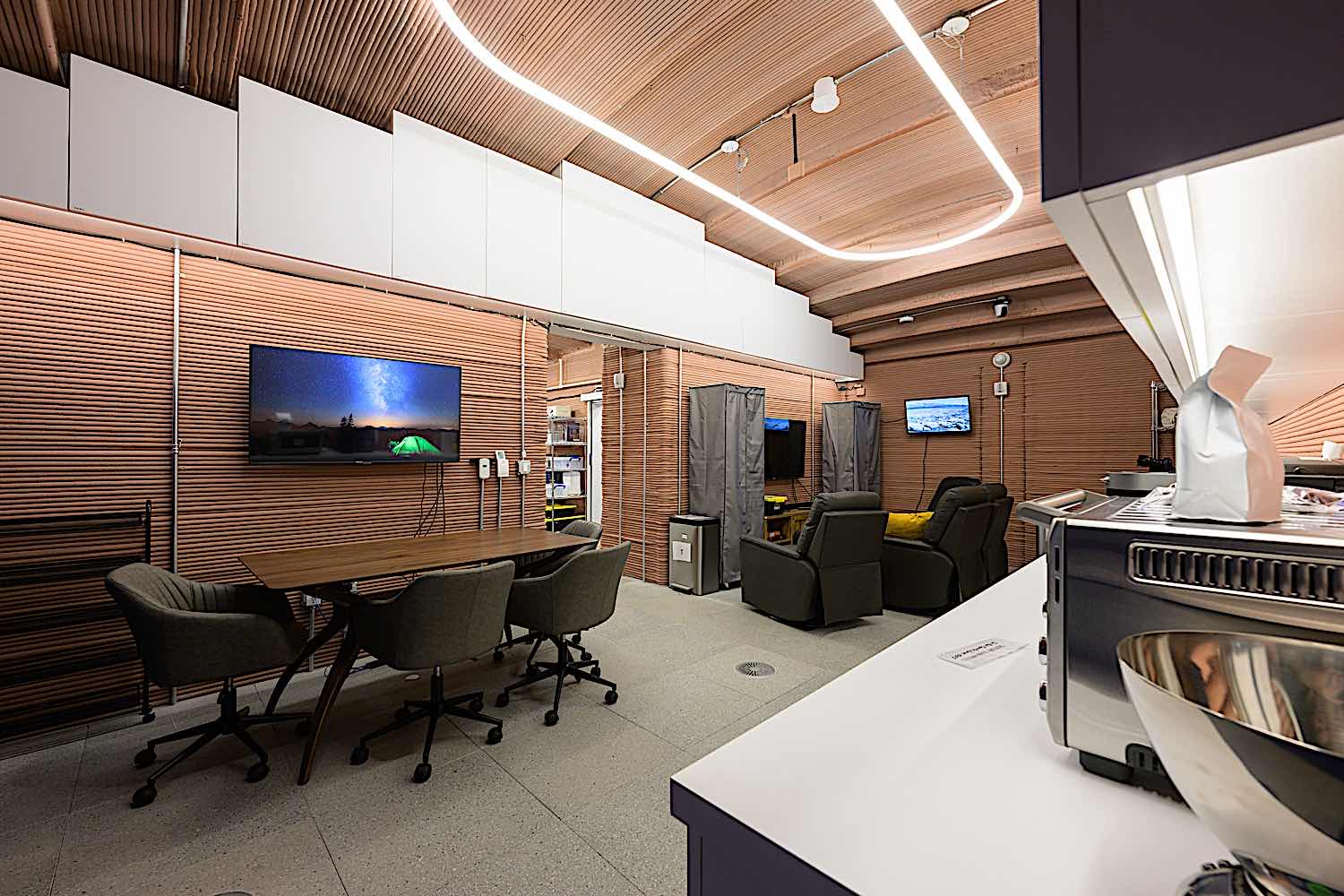
LOCATION: Bldg. 220 – CHAPEA
SUBJECT: Photography of Crew Health and Performance Exploration Analog (CHAPEA) facility
PHOTOGRAPHER: BILL STAFFORD
Sustainable Space Habitats: The Role of In-Space Construction
Imagine a dwelling beyond our planet, crafted not from materials ferried through the gravity well but assembled or even fabricated directly in space. This is the promise of in-space construction—a vision rapidly transitioning from the realm of science fiction to plausible reality. Creating sustainable habitats in space goes beyond merely establishing a physical location. It encompasses the ability to construct, maintain, and adapt these structures to ensure the long-term viability of human life away from Earth.
Harnessing In-Situ Resources
One cornerstone of sustainable space habitats is leveraging local materials, a practice known as in-situ resource utilization (ISRU). By using resources like lunar regolith or Martian soil, we can significantly reduce the dependency on Earth-sourced supplies. This not only slashes costs but also promotes the development of self-sufficient colonies.
Advanced Materials and Techniques
Emerging materials and innovative construction techniques are pivotal. Engineers are experimenting with materials that can withstand harsh space environments while being lightweight enough for transport if needed. For instance, sulfur-based concrete and basalt fiber composites, crafted from lunar or Martian materials, are being explored for their potential use in habitat construction. Additive manufacturing techniques, such as 3D printing, play a crucial role here, offering the flexibility to create complex structures on-site.
Adaptive and Resilient Designs
Space habitats must be resilient and adaptable to the unpredictable conditions of space. This includes modular designs that can be easily expanded or reconfigured as missions require, and smart systems that can autonomously manage and repair the habitat’s environment. Advances in artificial intelligence enable these habitats to monitor structural integrity, assess environmental conditions, and even undertake repairs, ensuring the safety and sustainability of the habitat over time.
Environmental Control and Life Support Systems (ECLSS)
Sustaining human life in space hinges on sophisticated Environmental Control and Life Support Systems. These systems must recycle air, water, and waste with minimal reliance on Earth. Innovations in closed-loop life support, bioregenerative systems, and efficient energy management are all vital components in creating a self-sustaining habitat. Plants and other biological systems are being integrated into habitats, not just for food production but also for oxygen generation and carbon dioxide removal.
The Human Factor
The human element cannot be overstated. The psychological and physiological well-being of astronauts is paramount. Thus, habitat designs incorporate elements that address these needs, such as artificial gravity, radiation shielding, and spaces designed to promote mental health. Establishing a home, not just a shelter, in space is key to the future of long-term space habitation.
The advancement of in-space construction technologies offers us the opportunity to redefine our approach to space exploration and habitation. By building sustainable habitats, we are laying the groundwork for a future where humans can thrive beyond Earth, turning our aspirations of living among the stars into a tangible reality.