As humanity ventures further into the cosmos, the need for autonomous space exploration innovations becomes increasingly critical, particularly for long-duration space missions.
Bioregenerative and Sustainable Systems will be as important as Autonomous Vehicle support and maintenance.
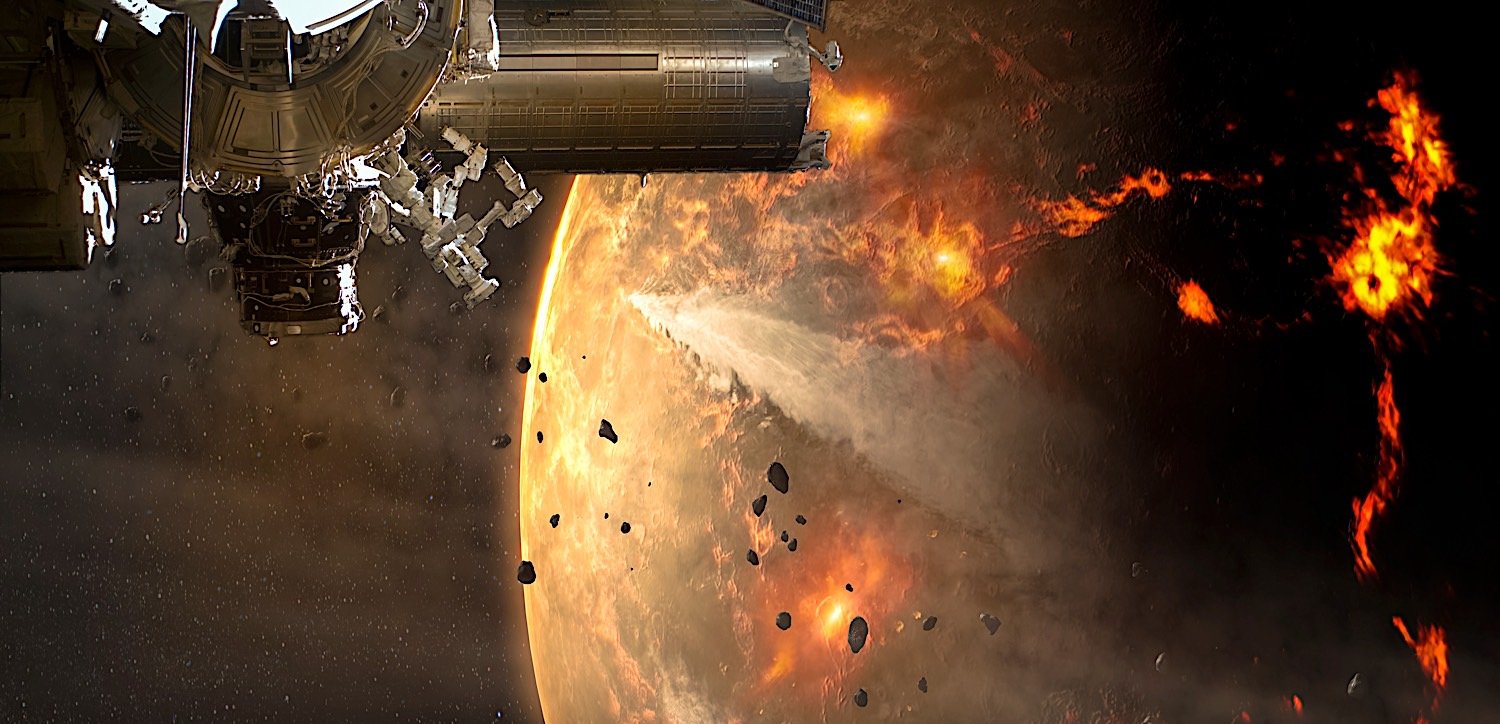
These missions, which are planned to last for months or even years, pose unique challenges that require advanced technologies capable of operating independently in the harsh environment of space. Whether manned or unmanned, these missions need to be autonomous and self-renewing for possibly years.
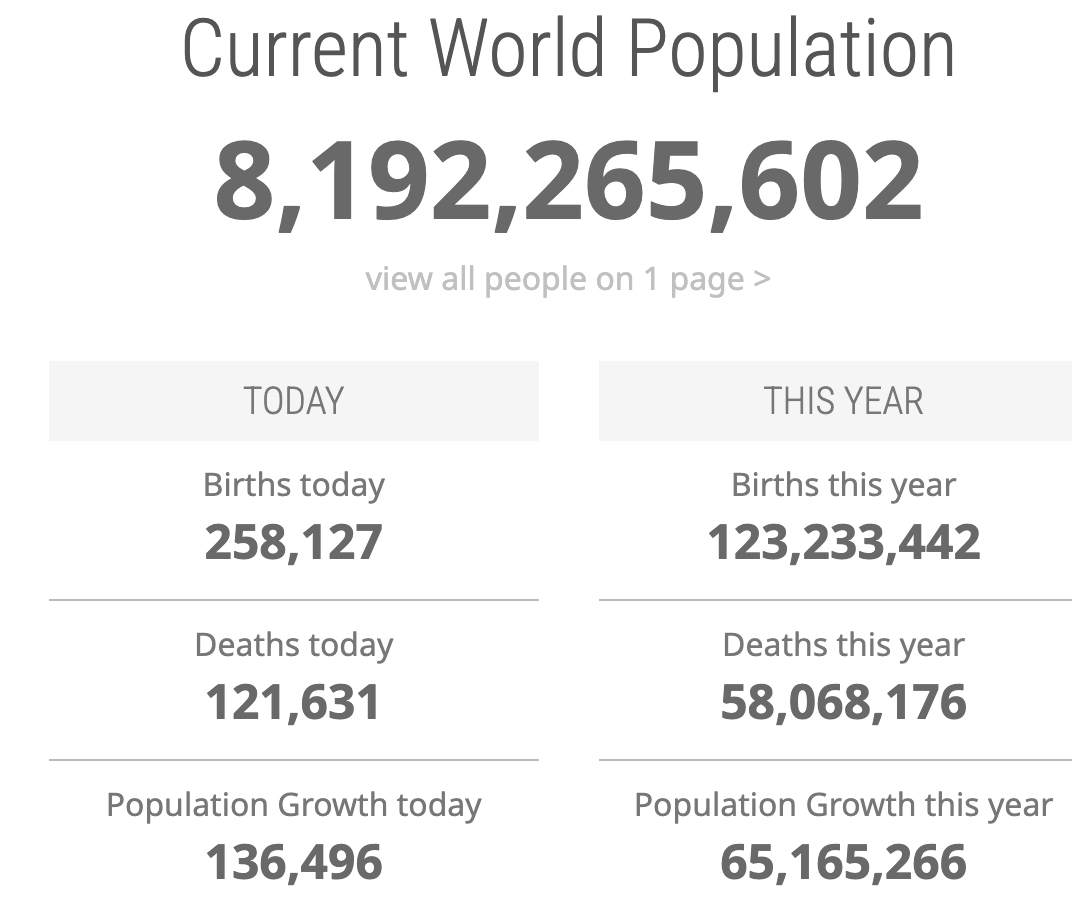
Is it Worth the Cost and Risk?
On the question of value — is it worth the risk and the extraordinary cost projections — the answer is a long-term yes. Space-mining, for example is projected to be a rich resource for centuries to come. Sustaining a population that doubles every twenty years or so (projected) will not be feasible long term without investing in space exploration and mining.
In the time it took to research and draft this feature, the world population clock grew by 110,588 net (after 46000 deaths). (This represents half a day in real time.)
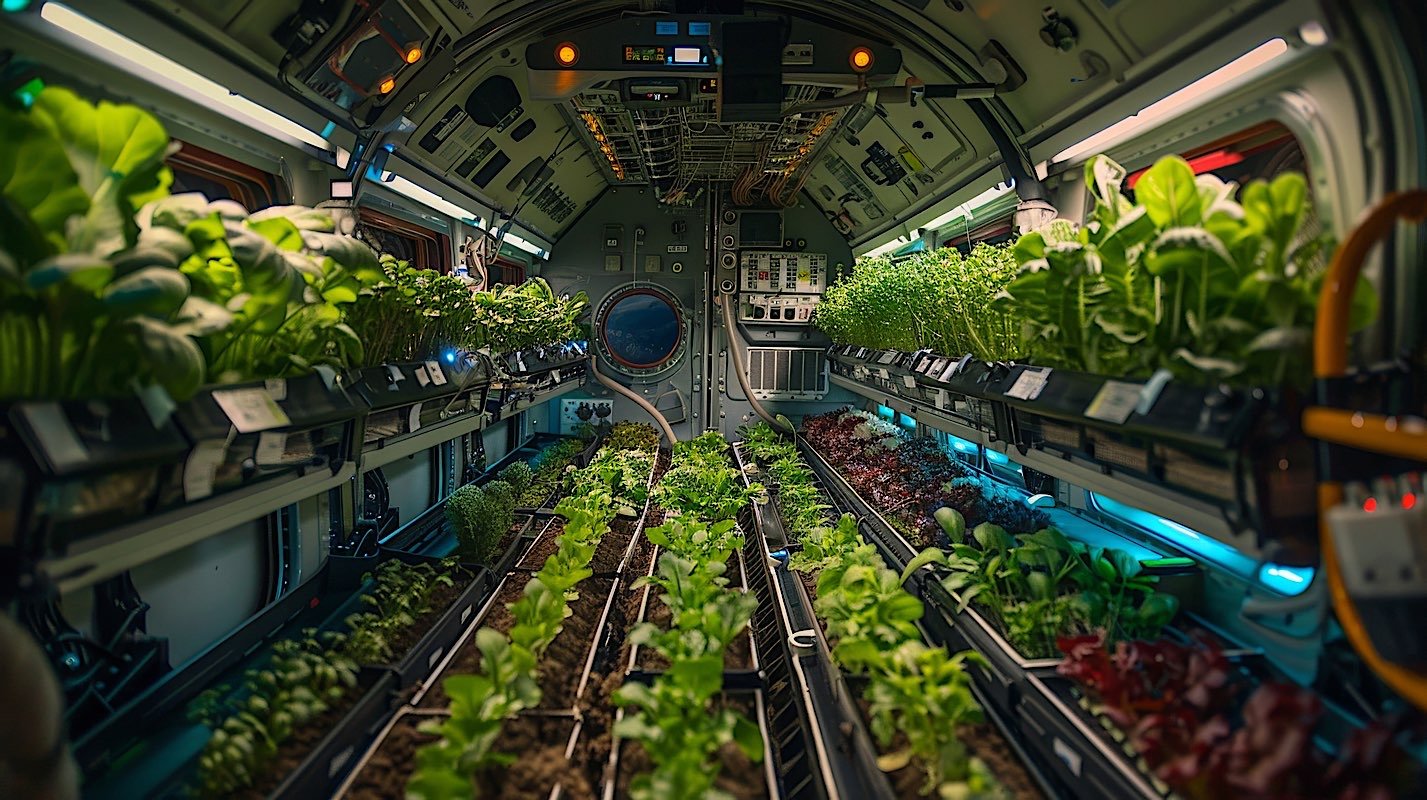
Stoichiometric model of a fully closed bioregenerative life support system
Bioregenerative life support is essentially a full sustainable life support system that can fully regenerate itself perpetually.
The system is designed to recycle resources completely, making it self-sustaining. It focuses on the chemical processes that occur within the system to maintain balance and support life. The goal is to create an environment that can support living beings without needing outside resources.
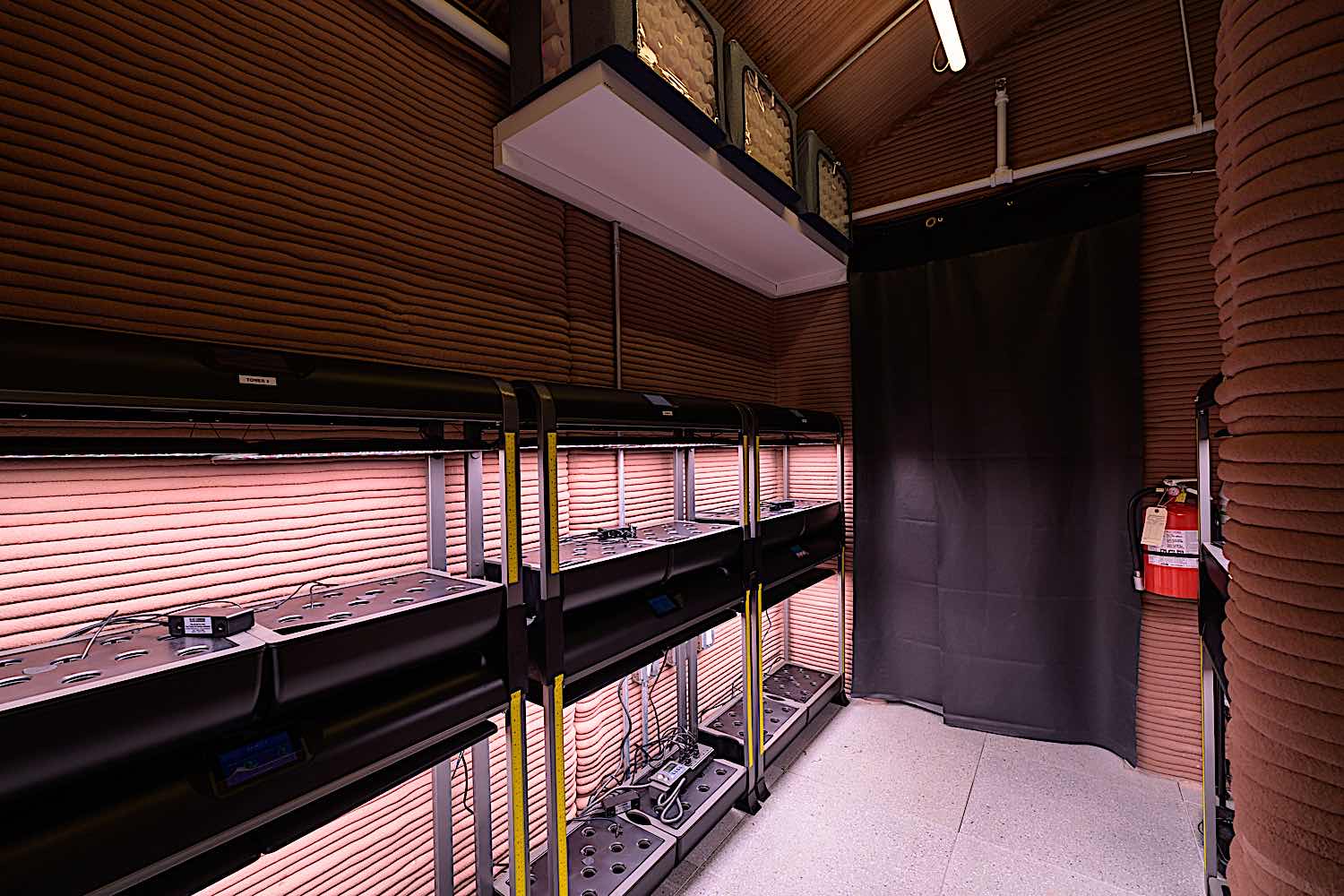
In a paper by A. Vermeulen, Alvaro Papic, Igor Nikolic, they summarized:
“Bioregenerative life support systems (BLSS) are vital for long-duration and remote space missions to increase mission sustainability. These systems break down human waste materials into nutrients and CO2 for plants and other edible organisms, which in turn provide food, fresh water, and oxygen for astronauts. The central idea is to create a materially closed loop, which can significantly reduce mission mass and volume by cutting down or even eliminating disposable waste. “
In itself, this proposition is already staggeringly challenging, but the authors of the paper add the ultimate goal of “missions without material resupply” — a reality for deep space travel:
“In most BLSS studies only a fraction of the resources, such as food, are provided by the system itself, with the rest taken on board at departure or provided through resupply missions. However, for autonomous long-duration space missions without any possibility of resupply, a BLSS that generates all resources with minimal or no material loss, is essential.”
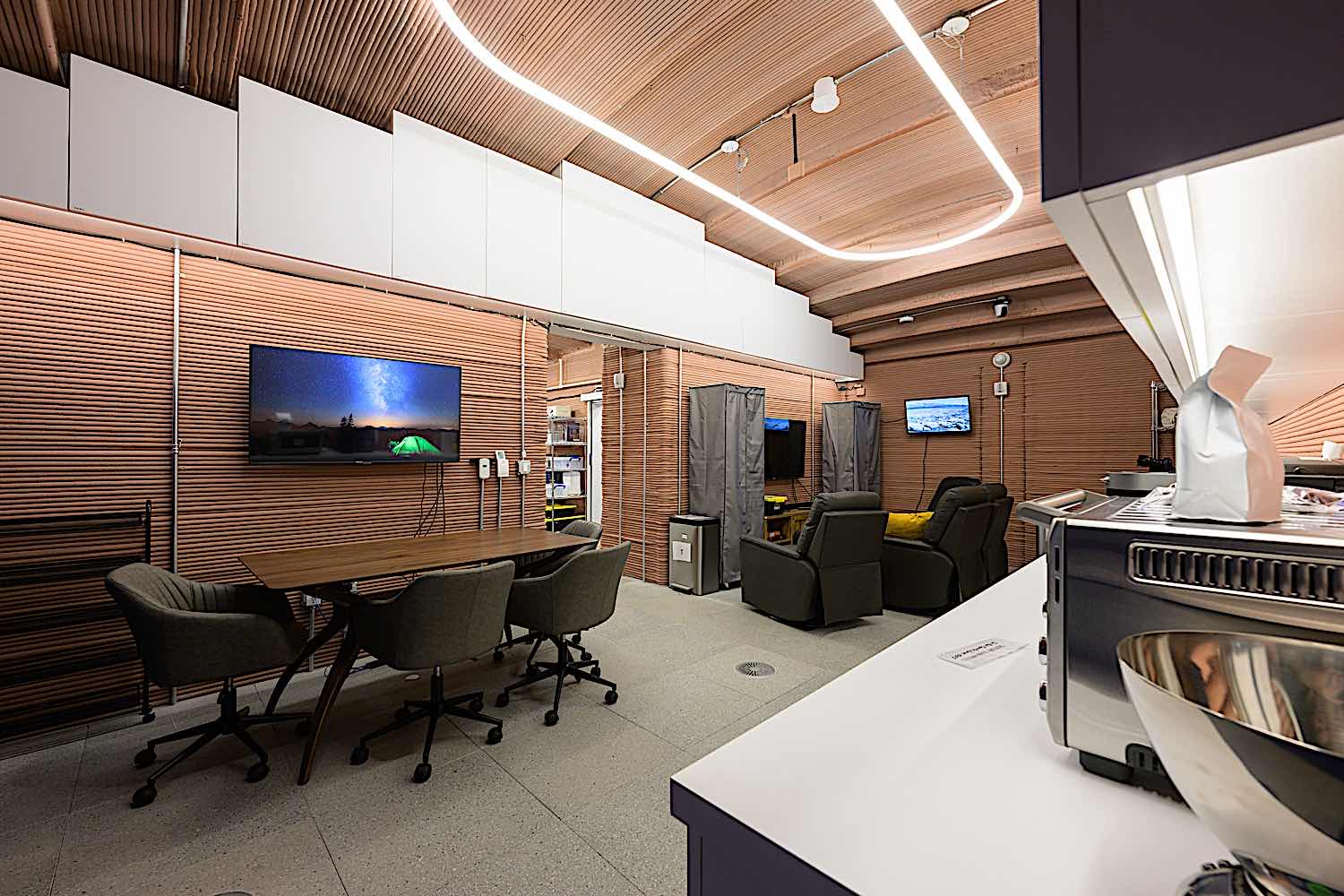
LOCATION: Bldg. 220 – CHAPEA
SUBJECT: Photography of Crew Health and Performance Exploration Analog (CHAPEA) facility
PHOTOGRAPHER: BILL STAFFORD
Robotics and Their Impact on Mission Sustainability
Robotics is another cornerstone of autonomous innovations in space exploration. Robotic systems can perform a variety of tasks, from maintenance and repairs to scientific experiments, all while minimizing the need for human presence in potentially hazardous environments — and in particular in the future when mining is projected to be a large space enterprise.
For example, rovers equipped with advanced sensors and tools can explore planetary surfaces, collect samples, and conduct experiments autonomously. This capability not only ensures mission sustainability by reducing the risk to human life but also allows for the continuous gathering of valuable scientific data. Thus, the role of robotics in supporting long-duration missions directly connects to the thesis by enhancing crew safety and mission effectiveness.
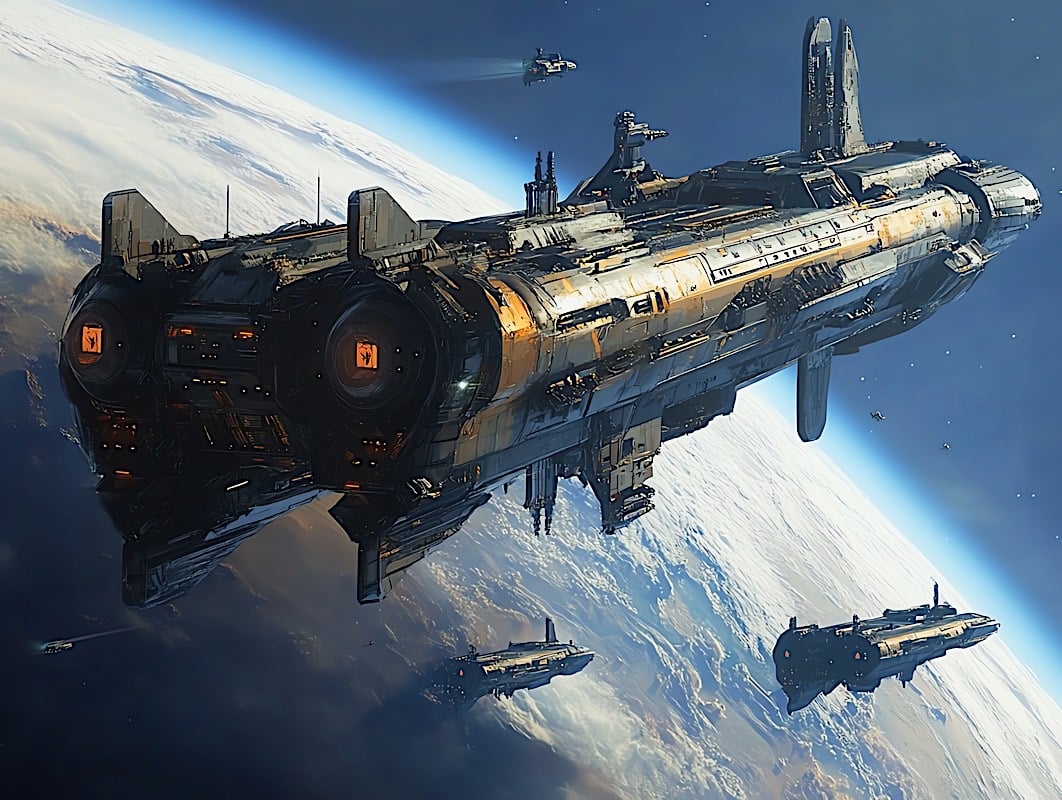
Advanced Life Support Systems for Long-Duration Missions
Advanced life support systems are crucial for sustaining human life during extended space missions. According to research “Reliability of life support systems depending on the degree of their biologisation.
Citing the example of Mars, the author of “Reliability of Life Support Systems” Sergey Glukhikh wrote:
“Reliable technologies of life support systems (LSS) of autonomous transport systems (ATS) mastered in space missions can be fully or fragmentarily used in missions of other habitats. The reliability of any long-term manned missions depends on the degree of LSS autonomy, the level of its biologisation, and the efficiency of integrated closed biotechnological cycles. With the help of unmanned interplanetary vehicles, the presence of water reserves on Mars has been proved, the Mars atmosphere has been studied, and methane, the origin of which is yet to be discovered, has been discovered, all of which will undoubtedly contribute to the exploration of the planet. This indicates the possibility of organising human life on Mars and contributes to the preparation of a manned mission with the mission of thorough exploration of the planet.”
Innovations in closed-loop life support systems, which recycle air, water, and waste, are essential for reducing reliance on resupply missions from Earth. Technologies such as bioregenerative systems, which use plants to produce oxygen and food while filtering carbon dioxide, can create a self-sustaining environment for astronauts. This not only addresses the logistical challenges of long missions but also enhances the psychological well-being of the crew by providing a semblance of Earth-like living conditions. Therefore, the development of advanced life support systems is integral to the thesis, as it ensures the health and safety of crew members during prolonged space exploration.
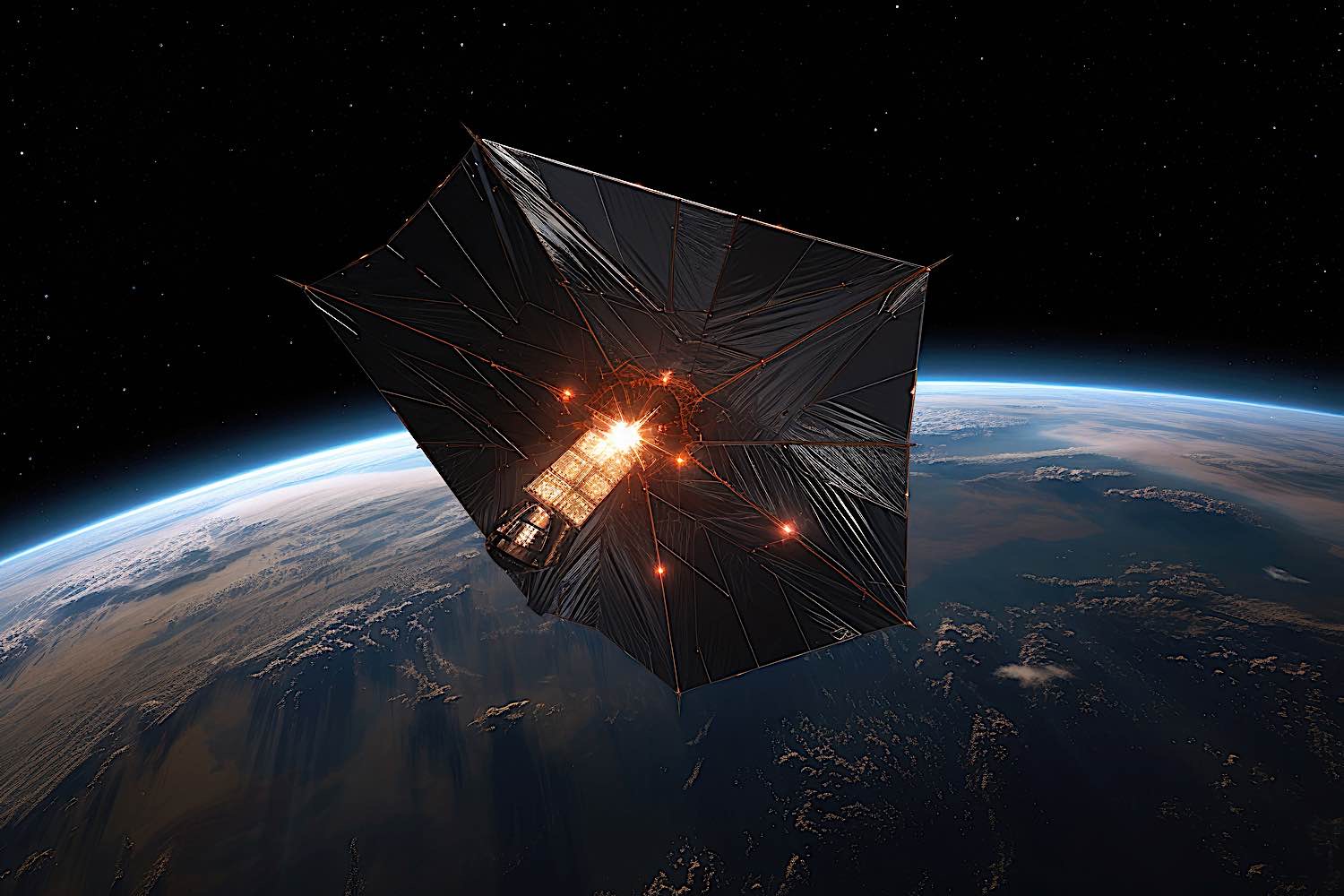
Completely Self-Sufficient Missions
The advancement of autonomous space exploration innovations is vital for the success of long-duration self-sufficient missions. The integration of regenerative systems, robotics, advanced life support systems, and maintaining robotic systems, is essential for operational efficiency and mission viability.
As we look to the future of space exploration, the implications of these technologies raise important questions about the sustainability of human presence in space and our ability to explore asteroids for minerals, and distant worlds for possible colonization as our populations doubles and double again.
The continued investment in and development of these innovations will be crucial as we strive to unlock the mysteries of the universe and expand our presence beyond Earth.
References
- Sergey Glukhikh. (2023). Reliability of life support systems depending on the degree of their biologisation. In E3S Web of Conferences. https://doi.org/10.1051/e3sconf/202343101020
- A. Vermeulen, Alvaro Papic, Igor Nikolic, & Frances M. T. Brazier. (2023). Stoichiometric model of a fully closed bioregenerative life support system for autonomous long-duration space missions. In Frontiers in Astronomy and Space Sciences. https://doi.org/10.3389/fspas.2023.1198689
- I. Kabashkin, & Sergey Glukhikh. (2023). Life Cycle Cost Model for Life Support Systems of Crewed Autonomous Transport for Deep Space Habitation. In Applied Sciences. https://doi.org/10.3390/app13148213
- I. Kabashkin, & Sergey Glukhikh. (2023). Closed biotechnological cycles for transport life support systems in deep space exploration. In E3S Web of Conferences. https://doi.org/10.1051/e3sconf/202338905028
- I. V. Markin, E. S. Shchelkanova, R. A. Volodyashkin, E. A. Zhurbin, & A. Fisun. (2021). Prospects for Creation of Autonomous Life Support Complexes Using Biological Systems for Arctic and Far North Conditions. In Disaster Medicine. https://doi.org/10.33266/2070-1004-2021-3-73-80